research description
The Clark research group focuses on the applications of organometallic chemistry to organic synthesis. Organometallic complexes are used as catalysts to mediate organic reactions that either cannot be achieved without a metal, or are much more efficient and practical with the metal present. The goals of the research projects are to develop new catalysts that are synthetically useful and ultimately to apply the newly developed reaction to a synthetic target. Projects in my group range from synthetic organometallic chemistry (design of new transition metal catalysts) to new organic reaction development using transition metal catalysts. The focus of our research is on the introduction of boron substituents into organic molecules. New methods to incorporate boron into organic molecules are valuable synthetic tools to develop because the C–B bond is so versatile and can be converted into C–C, C–O, C–N, and other C–X bonds. Therefore, new methods to introduce boron into organic molecules will simplify the synthesis of complex targets such as pharmaceutical drugs, natural products, organic materials, and organocatalysts. Pharmaceuticals containing boron are also recieving significant attention in recent years, leading to several FDA-approved drugs for the treatment of cancer and bacterial fungal infections.
Undergraduate Student-Focused Research Program
The University of San Diego is a Predominantly Undergraduate Institution (PUI) and the Department of Chemistry and Biochemistry does not have graduate students. The majority of the work done in the Clark research group is by undergraduate students, providing valuable hands-on training in synthetic organic and organometallic chemistry. This experience provides students with added confidence and ability to succeed in a career in chemistry or biochemistry or in graduate school. Students take ownership of their research project and are slowly guided toward independence. Professor Clark has mentored over 60 undergraduate students, 9 high school students, and 4 post-doctoral research associates since 2007. Students have gone to and excelled in graduate programs such as UCLA, UC Irvine, Colorado State University, UNC Chapel Hill, Scripps Research Institute, University of Michigan, UCSC, UCSB, Rutgers University, Oregon State University, and University of Delaware. Many students have also gone straight into the biotechnology workforce, medical school, and other career paths that utilize their science background. Dr. Clark welcomes applications from undergraduate students from within and outside of USD. Please contact him by email (clarkt@sandiego.edu). Professor Clark also mentors postdoctoral research associates that are interested in a career at a PUI when funding is available. Please contact Professor Clark for additional information on opportunities in his research group.
Copper-Catalyzed Diboration or Carbonyl Compounds
Recently, the applications of alpha-heteroatom-substituted boronate esters in asymmetric synthesis and as pharmaceutical targets have necessitated a general way to form these intermediates. Our group is interested in using copper-catalyzed diboration reactions to generate alpha-hydroxy-substituted boronate esters (Scheme 1). Thus far, we have developed improved conditions for the diboration of aldehydes and ketones based on the copper catalyst originally reported by Sadighi and co-workers, providing the first example of tertiary alpha-hydroxyboronate esters.1 We have also made progress in developing new methods to utilize the alpha-hydroxyboronate esters as synthetic intermediates.
Scheme 1. The Copper-Catalyzed Diboration of Aldehydes and Ketones
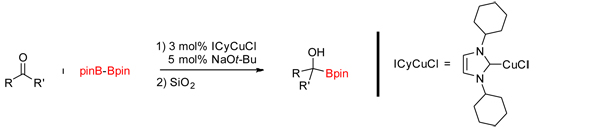
Scheme 2. Acid-Catalyzed Elimination of Alpha-Hydroxyboronate Esters
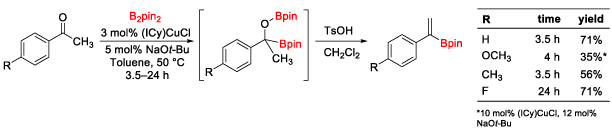
The synthetic utility of ketone diboration products was demonstrated in the acid-catalyzed elimination to generate 1,1-disubstituted (Scheme 2) and trisubstituted vinylboronate esters (Scheme 3) with good control of the olefin geometry in most cases. The reaction conditions are simple and easily reproduced through a two-step, one-flask reaction sequence. The vinylboronate esters have been subjected to Suzuki-Miyaura coupling conditions with aryl halides to provide a variety of styrene derivatives (not shown).2
Scheme 3. Synthesis of Trisubstituted Vinylboronate Esters
rs
We have also utilized aldehyde diboration products in the Matteson homologation reaction. Initially it was thought that the alpha-hydroxy substituent would require protection prior to homologation, but direct homologation with excess LiCH2Cl was developed, providing beta-hydroxyboronate esters in good to high yields in two steps from the aldehydes (Scheme 4).3 We are currently working to expand this homologation reaction to more complex homologating agents. We recently reported the use of allyl chloride as a homologating agent, for example.4 This project has been funded by the Research Corporation for Science Advancement and the National Institutes of Health.
Scheme 4. Diboration/Homologation Sequence with Aldehydes 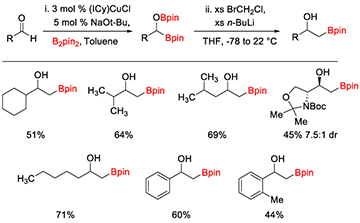
Iridium-Catalyzed C-H Borylation Reactions
The second major project in our research group is on substrate-directed C–H borylation reactions using iridium catalysts. The original focus was on the development of a bifunctional ligand that could direct the C–H borylation reaction, which ultimately resulted in a ligand (picolylamine) that induces directed C–H borylation by partial dissociation of one arm of a diamine ligand (Scheme 5).5
Scheme 5. Amine-Directed Ortho C-H Borylation Reaction 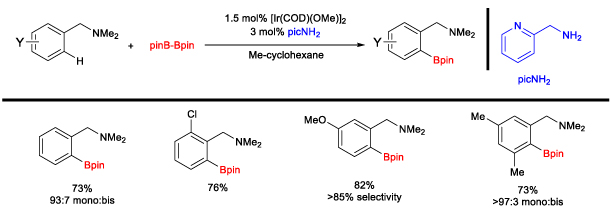
The catalyst efficiency and selectivity were improved (for monoborylation over bisborylation) through a comprehensive study of bidentate ligands with an emphasis on diamino ligands. The role of each nitrogen was explored to probe the partial dissociation of one arm of the ligand. Local steric effects were also examined, demonstrating a significant influence on borylation selectivity. Ultimately, it was found that the diamine bite angle played a significant role in both selectivity and reactivity. A sub-set of ligands is summarized in Scheme 6, demonstrating the steric, electronic, and bite angle effects on conversion and selectivity.
Scheme 6. Effect of Ligands on C-H Borylation Selectivity and Conversion
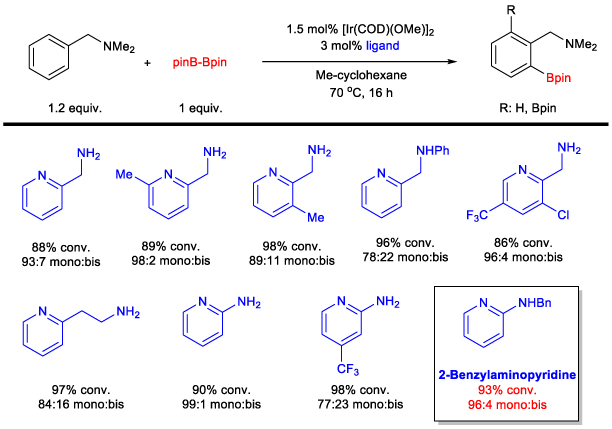
2-(N-Benylamino)pyridine was found to have optimal reactivity and selectivity.6 These studies are central to our initial understanding of the reaction mechanism. We are now working on a detailed mechanistic study of this catalytic reaction.
We have also focused some attention on the synthetic utility of the resulting arylboronate esters. So far, we have developed the Suzuki-Miyaura coupling reaction7 and the Chan-Evans-Lam amination8 and etherification9 of the borylated benzylic amines described above (Scheme 7). The resulting products are embedded in a number of biologically active compounds (Anacetrapib and Tubocurarine Chloride are shown). For the amination and etherification reactions, these are one of only a handful of Chan-Evans-Lam reactions that can be accomplished directly on the boronate ester rather than the boronic acid. We are currently examining ways to make this direct coupling more general and to understand the improved reactivity with the benzylic amine present. This project has been funded by the American Chemical Society-Petroleum Research Fund and a National Science Foundation Early CAREER Award and an NSF RUI grant.
Scheme 7. Synthetic Utility of Amine-Directed C-H Borylation
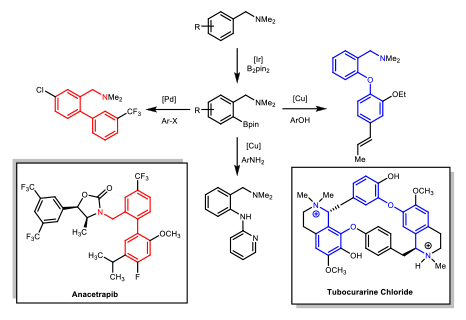
Synthesis of Ambiphilic Phosphine Boronate Esters
A recent direction in our group is focused on phosphine-directed C-H borylation reactions which emerged from our studies of the amine-directed C–H borylation reaction described above. The results obtained with the ligand screen (Scheme 6) were used to begin looking at additional directing groups. It was found that phosphines were capable of directing the C–H borylation of arenes. Interestingly, picolylamine was an ineffective ligand to mediate this transformation, but 2-benzylaminopyridine provided moderate conversion. Optimal yields were obtained, however, without the addition of an external ligand. The reaction conditions have been optimized and the resulting phosphine boronate esters were protected as the borane complex to facilitate isolation of the air-sensitive products (Scheme 8). X-ray crystal structures of several protected phosphines have been obtained to confirm the site of borylation. Deprotection conditions have been established to provide the phosphine boronate esters.10
Scheme 8. Phosphine-Directed C-H Borylation/Borane Protection
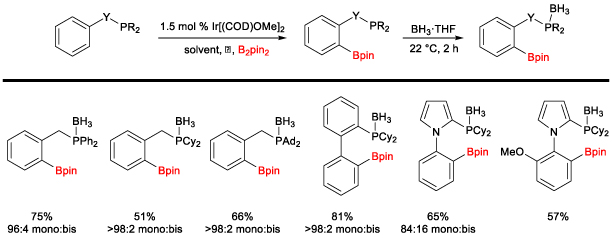
The scope of the reaction was recently expanded by identifying a cationic iridium complex that could catalyze the phosphine-directed C-H borylation. The new system resulted in a wide variety of phosphines that were readily borylated.11 Several phosphines could also be polyborylated in good yields. Deprotection of the phosphines boronates provides interesting ambiphilic phosphine boronates, potential organocatalysts that are currently being examined.
Scheme 9. Phosphine-Directed C-H Borylation with Borylation with Cationic Iridium Complex
References:
(1) McIntosh, M. L.; Moore, C. M.; Clark, T. B. Org. Lett. 2010, 12, 1996.
(2) Guan, W.; Michael, A. K.; McIntosh, M. L.; Koren-Selfridge, L.; Scott, J. P.; Clark, T. B. J. Org. Chem. 2014, 79, 7199.
(3) Moore, C. M.; Medina, C. R.; Cannamela, P. C.; McIntosh, M. L.; Ferber, C. J.; Roering, A. J.; Clark, T. B. Organic Letters 2014, 16, 6056.
(4) Meyer, G. F.; Nistler, M. A.; Samoshin, A. V.; Thane, T. A.; Ferber, C. J.; McManus, B.; O'Neil, G. W.; Clark, T. B. Tetrahedron Letters 2020, 61, 152082.
(5) Roering, A. J.; Hale, L. V. A.; Squier, P. A.; Ringgold, M. A.; Wiederspan, E. R.; Clark, T. B. Org. Lett. 2012, 14, 3558.
(6) Hale, L. V. A.; McGarry, K. A.; Ringgold, M. A.; Clark, T. B. Organometallics 2015, 34, 51.
(7) Hale, L. V. A.; Emmerson, D. G.; Ling, E. F.; Roering, A. J.; Ringgold, M. A.; Clark, T. B. Org. Chem. Frontiers 2015, 2, 661.
(8) McGarry, K. A.; Duenas, A. A.; Clark, T. B. J. Org. Chem. 2015, 80, 7193.
(9) Marcum, J. S.; McGarry, K. A.; Ferber, C. J.; Clark, T. B. J. Org. Chem. 2016, 81, 7963.
(10) Crawford, K. M.; Ramseyer, T. R.; Daley, C. J. A.; Clark, T. B. Angew. Chem., Int. Ed. 2014, 53, 7589.
(11) Wright, S. E.; Richardson-Solorzano, S.; Stewart, T. N.; Miller, C. D.; Morris, K. C.; Daley, C. J. A.; Clark, T. B. Angew. Chem., Int. Ed., 2019, 58, 2834-2838. |